When the Greeks observed the errant lights in the sky—which they named planets—they probably never envisioned that, one day, we could actually reach the surfaces of some of them to explore their bowels.
In May 2018, NASA launched the mission InSight: Interior exploration, Seismic Investigations, Geodesy, and Heat Transport. Upon its successful arrival on the surface of Mars in November 2018, the lander has been working tirelessly, listening to the inner “voices” of Mars.
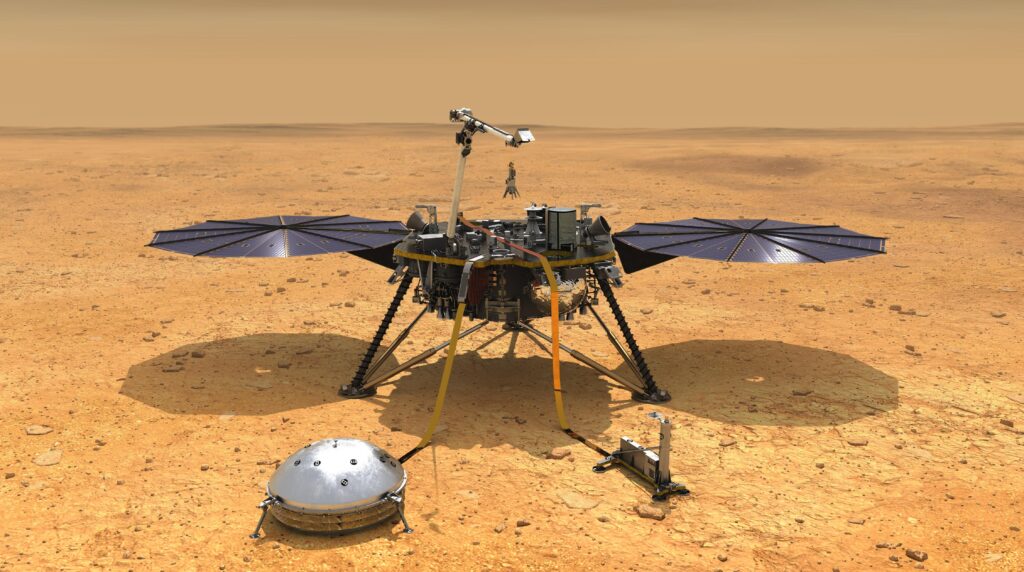
After almost a thousand Martian days, scientists are ready to announce their preliminary findings on Mars’ layers: crust, mantle, and core. The results were published yesterday in three articles in the July 23 issue of Science.
The researchers investigated the data produced by the instrument SEIS: Seismic Experiment for Internal Structure of Mars, which can detect waves arising from quakes inside the planet.
How do such quakes help scientists scrutinize the interior of a planet?
Discovering the structure of rocky planets by listening to them
Here on Earth, geophysicists use several methods to explore the inside of the planet without drilling. One of the most popular methods is the seismic survey, which consists of detecting vibrations coming from inside the planet.
Similar to ultrasound scans that allow an obstetrician to see images of the fetus inside the mother’s body, seismic methods allow geophysicists to image the inside of the Earth to obtain valuable information about its structure and composition.
Quakes are vibrations traveling inside the planet as waves or seismic waves. When seismic waves pass from one material to another, part of them gets refracted (bent), just like light entering a glass of water, while the remaining waves get reflected in the boundary between the two materials. Depending on the nature of the layers, i.e., whether they are rocks or liquified material, seismic waves speed up or slow down. Instruments detect these changes in the waves, and geophysicists use this data to image the Earth’s interior.
Changes in the media disturb the seismic wave’s components: S-waves and P-waves. P-waves resemble a spring, while S-waves cause changes in the material’s shape without changing its volume. S-waves need elastic materials to propagate and therefore cannot travel in fluids, which are inelastic: only the P-waves survive propagation through liquids. When the seismic waves leave the liquid, information about the S-waves is partially lost: the detector only sees P-waves. This way, geologists know the waves have passed through a fluid.
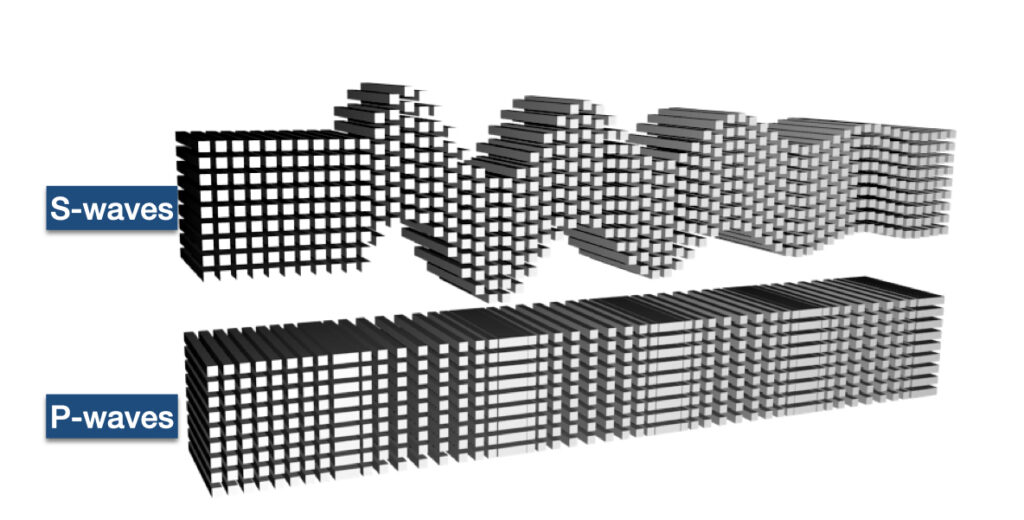
Primary waves (P-waves) are compressional waves that are longitudinal in nature. P-waves are pressure waves that travel faster than other waves through the earth to arrive at seismograph stations first, hence the name “Primary”. Secondary waves (S-waves) are shear waves that are transverse in nature. Following an earthquake event, S-waves arrive at seismograph stations after the faster-moving P-waves and displace the ground perpendicular to the direction of propagation
Temperature gradients also affect the behavior of seismic waves, pretty much like how the refractive index of air changes on a hot day. These changes provide the scientists valuable information about the interior of the place they investigate.
So, if scientists can listen to the bowels of the Earth, why not of another rocky planet?
Understanding Mars’ interior in layers
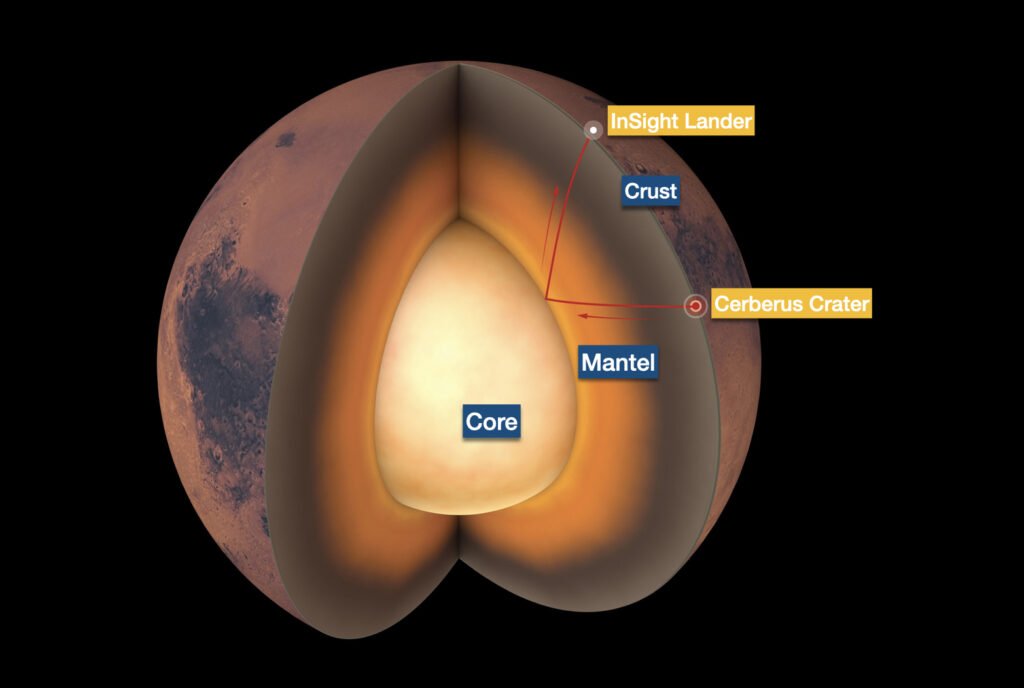
Seismic waves tell us about the properties and boundaries of a planet’s interior. Shear waves that travel from a marsquake and reflect off the iron-nickel core are detected by the Insight seismometer and give us an estimate of the core size. The strength of the reflected waves shows the core is in a liquid state, which shear waves cannot propagate through. Credit: Chris Bickel/Science.
The crust
Brigitte Knapmeyer-Endrun, University of Cologne, Germany, and collaborators investigated the thickness and structure of the Martian crust. They found evidence of a thick multi-layered structure with either two or three interfaces.
Their models showed “robust and consistent depths of the two shallowest interfaces” under the Insight landing site. The first layer would be around 6 to 11 kilometers, whereas a second interface would be 15 to 25 kilometers deep.
Extrapolating their model to the whole planet, the scientists estimated an average thickness of the Martian crust between 24 and 72 kilometers.
The mantle
Amir Khan from the Swiss Federal Institute of Technology in Zürich-ETH and collaborators investigated the upper mantle structure of Mars. Listening as deep as 800 kilometers inside Mars, they collected data from vibrations coming in less than one wave per second (< 1 Hz).
Focusing on eight clear events, the results suggest that Mars has a thick lithosphere that lies close to 500 km below the surface. The lithosphere is defined by the crust plus the upper mantle. On Earth, it is only 80km thick on average.
Seismic investigations also shed light on the local geochemistry. Amir Khan explained further their findings:
“Our study has shown that when you compare the seismic results on thickness of the crust and lithosphere and upper mantle thermal gradients with thermal evolution calculations that model the evolution of Mars over ~4.5 billion years, the crust contains more radioactive elements than we previously thought. Earlier determinations were based on gamma-ray spectrometer data obtained from the Mars Global Surveyor (MGS) satellite in orbit about Mars about 10 years ago. But MGS measured the radioactive element content of the surface material, whereas we’ve been looking inside the crust. That’s where the differences come from. This means that the crust is different at depth from what is seen on the surface (at least from the point of view of radioactive element content).”
The core
Simon Stähler from the Swiss Federal Institute of Technology in Zürich-ETH and collaborators investigated reflected seismic waves from what they interpret as the Martian core. These waves reveal a huge liquid iron-nickel core with a radius of nearly 1,830 kilometers, which begins roughly halfway between the surface and the center of the planet.
Their article explains that “a large core with a radius range between 1,800 and 1,900 km would be enriched in light elements“.
These other elements “are probably Sulfur (<10%), Oxygen (<2%), Hydrogen and Carbon (both <1%). But this combination is not enough to create such a light core. So there needs to be something about the composition of Mars that we do not understand yet,” Simon Stähler said.
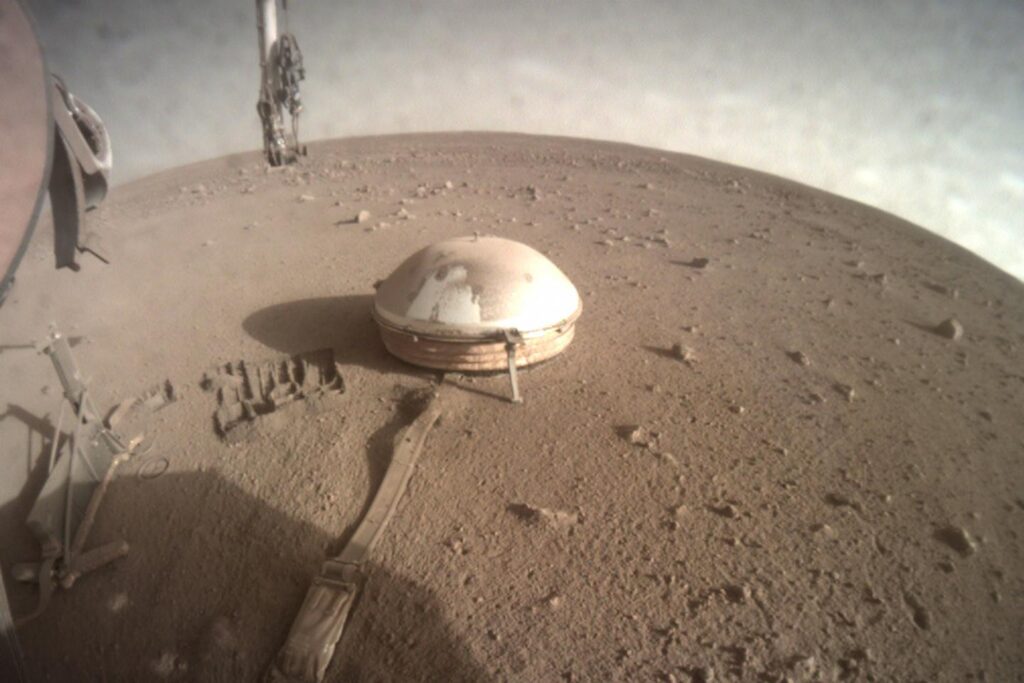
Marsquakes, meteorites, and tectonic activity on Mars
Mars is, in many senses, a rocky dead planet. It does not have tectonic plates moving around like here on Earth. So, where do all these marsquakes detected by InSight come from? What kind of tectonic activity does Mars have?
Brigitte Knapmeyer-Endrun explained:
“Marsquakes, like [many] earthquakes, are caused by faults—rock fractures created by stress in the outer brittle layer of the planet. Faults still form in the Martian crust due to stresses caused by the slight shrinking of the planet due to cooling. So, there is tectonic activity on Mars, also evidenced by offsets along faults that have been observed from orbit.”
Science Mars has a very thin atmosphere, lots of meteorites do impact its surface. However, InSight has not yet detected “any meteorite impacts in the seismic data,” Amir Khan said, and clarified:
“We have already seen locations on Mars where images showed that within a ~1 year an impact has occurred. However, we have not been able to locate these impacts in the seismic data. Probably because the impacts are too small and too far away.”
Cerberus crater
According to Birgitte Knapmeyer-Endrun, the most significant quakes recorded by InSight appear to come from Cerberus Fossae, which is “a faulted region with traces of recent volcanic activity.”
Scientists consider Cerberus Fossae as the youngest Mars region. It shows activity from the past 25 million years, in contrast with the rest of the planet’s surface, which is billions of years old and contains large fissures filled with 10 million years old lava. According to Birgitte Knapmeyer-Endrun:
“Orbiting spacecraft have spotted the tracks of boulders that may have been shaken down steep slopes by marsquakes. This area was in fact identified as a possible source region for marsquakes before the mission. So we think at least one group of marsquakes, the low-frequency family of events that are used in all of the three studies on the interior, are indeed tectonic events.”
Previous studies showed that much of Mars’ crust formed early in the planet’s history and was accompanied by substantial volcanism. But today, the God of war is actually a very pacific planet.
Simon Stähler is optimistic about the future of the investigation:
“We will operate for another year at least and this is the time when the weather is most favourable to observe quakes (because there is little wind that rattles the lander and creates noise). So we hope that this year will give us a lot more data. Also, we hope to detect quakes from the other side of the planet, of which the waves propagate through the core, so that we learn about the speed of waves inside. So far we only got reflections from outside.”
Studying Mars’ internal structure improves our understanding of how it was formed and evolved through time. Moreover, it may provide clues on the early formation of rocky planets in the inner solar system—Mercury, Venus, Earth, and Mars— more than 4 billion years ago, as well as of rocky exoplanets.
References
- Amir Khan, et al. (2021). Upper mantle structure of Mars from InSight seismic data. Science 373(6553), 434-438. DOI: 10.1126/science.abf2966
- Brigitte Knapmeyer-Endrun, et al. (2021).Thickness and structure of the martian crust from InSight seismic data. Science 373(6553), 438-443. DOI: 10.1126/science.abf8966
- Simon Stähler. (2021). Seismic detection of the Martian core. Science 373(6553), 443-448. DOI: 10.1126/science.abi7730
- Tony Greicius. (April 1, 2021). NASA’s InSight Detects Two Sizable Quakes on Mars. NASA news.
- L. T. Elkins-Tanton, Annu. Rev. Earth Planet. Sci. 40, 113–139 (2012).
- M. Grott et al., Space Sci. Rev. 174, 49–111 (2013).
Scientists interviewed
Dr. Brigitte Knapmeyer-Endrun, Faculty of Mathematics and Natural Sciences Institute of Geology and Mineralogy, University of Cologne, Germany.
PD. Dr. Amir Khan, Department of Earth Sciences, Institute of Geophysics, Swiss Federal Institute of Technology in Zürich-ETH, Switzerland.
Dr. Simon Christian Stähler, Department of Earth Sciences, Institute of Geophysics, Swiss Federal Institute of Technology in Zürich-ETH, Switzerland.